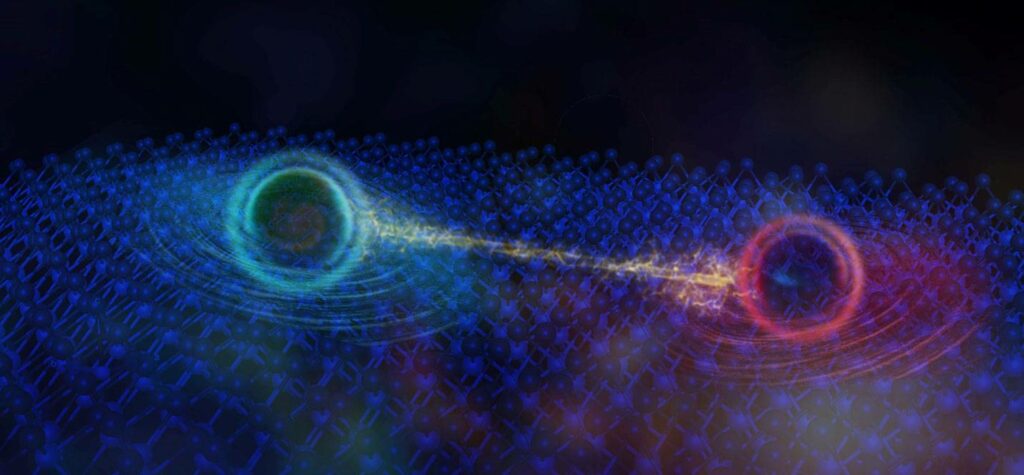
New Quantum Phenomenon Discovered in Heisenberg Magnets
A group of scientists at the MIT-Harvard Center for ultra-cold atoms; a collaboration between the Massachusetts Institute of Technology (MIT), Stanford University, and Harvard University have lately revealed the existence of unique helical spin states in Heisenberg quantum magnets. Their findings were published in a paper in Nature Physics, a monthly peer-reviewed scientific journal, which might have some important inferences for the simulation of spin-related physical methods and dynamics in quantum many-body systems.
Wen Wei Ho and Eunice (Yoo Kyung) Lee, two of the researchers who carried out the study, told Phys.org., that the primary objective behind this experiment was to examine the dynamics of quantum magnetism, as it highlights several of the technologies that are in use today, which also includes memory storage devices.
To illustrate quantum magnetism, it can be said that every elementary particle as carrying a spin that can point in various directions from each other. In this case, the two closest spins can exchange their locations through an intermediate state with both the particles at the same position.
Lee further explained that this idea was taken from the Heisenberg spin model, a simple textbook model, which was found in one dimension in an experimental platform using ultra-cold atoms. Usually, the spin pattern gets messed up over time if it is already aligned, and a random mixture of all spins can be observed pointing in different directions.
A recent study by a team of theoretical physicists at the University of Ljubljana and the University of Wuppertal suggested that the spins spiral along the chain of the X-Y plane and with a particular pitch known as “phantom helix states.”
Lee said that the Heisenberg model had been known for a hundred years now, so they are excited by these new ‘phantom helix states’ and are ready to observe them. To achieve this, they prepared a spin-helix state with a specific wavelength and then observed the contrast of the helix decayed over a certain period of time. In a recent paper, Lee and her colleagues also used calculations by Wen Wei Ho to know whether the decay rates they noticed were constant with theoretical predictions.
To achieve their aim, Lee and her team loaded ultra-cold lithium atoms into a 3D optical lattice, which was made by using three standing waves of extreme laser beams.
Lee said that the team initialized their spin helix by circling their magnets to the transverse plane, then winding it until they generated a transverse helix, which created a sinusoidal spin pattern. They extract the characteristic lifetime of these states by watching the decay rate. The helix’s winding angle with the least decay rate is the phantom helix state, which stays longer. They were also able to find out a way to determine the interaction anisotropy in their model.
Lee said that the Heisenberg model used in the experiment has various interaction strengths between the X-Y (transverse) and Z (longitudinal) directions. They can change this interaction anisotropy, Δ, by altering their magnetic field and making changes in the scattering lengths between particles. This parameter controls all the spin dynamics in this system.
Through this experiment, scientists were able to find out that phantom helix states can be used to measure this parameter directly, which is necessary to perform quantum simulations. These findings will be helpful in the future to increase the fidelity and reliability of various quantum simulations.
They also noticed that higher-order terms contributed to spin dynamics. According to the theory, the anisotropy rationally well when the interaction is small between two particles. This is the regime in which quantum magnetism is studied, as when interactions are larger, the model breaks down.
The findings collected by Lee and her team indicate that the theoretical models which describe a spin model are incomplete as they do not produce anisotropy estimations every time. As such, they plan to investigate more about the limitations of existing models in their future works and the mechanism behind the phantom helix states.
Their recent study also shows the potential connection between phantom helix states and quantum many-body scarring. Quantum many-body scars are a unique set of states in which a system’s ergodicity breaks down.
Many other teams of scientists have already discovered models that host quantum many-body scarring, so these models have been known to be challenging to identify in an experiment. On the other hand, the XXZ Heisenberg model created by Lee and her team defines one of the simplest many-body systems to identify, which additionally can support scarring.
This study of observing long-lived phantom helix states can contribute to various follow-up studies by other researchers around the world. Additionally, it could lead to the generation of more effective quantum simulation techniques.